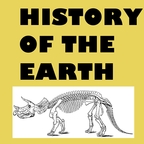
History of the Earth
Summary: 366 snapshots of earth history in the form of a perpetual calendar, with daily episodes for 2014 and weekly thereafter. Find all the posts at http://historyoftheearthcalendar.blogspot.com
- Visit Website
- RSS
- Artist: Richard I. Gibson
- Copyright: Copyright Richard I. Gibson
Podcasts:
The other day, when I was talking about the Atlantic and Gulf Coastal Plains of the United States, I mentioned sea-level changes that produced various locations for the Cretaceous shoreline. And I left it pretty much unexplained. These transgressions and regressions of the sea were mostly world wide, and there were at least five major transgressions or sea-level rises. An analysis of the facies, or rock types and structures in the rocks, suggests that the rate of sea-level rise could have been on the order of 10 to 90 meters per million years, which is obviously slow in human terms, as we wrestle with possible sea-level rises of a few meters in the next century, but it’s really a lot in a short time, in geological terms. At the end of the early Cretaceous, the Albian Age, the global sea level was probably similar to what it is today, but at times during the late Cretaceous, it was as much as a whopping 650 meters higher, although other estimates put the maximum at about 270 meters higher. That’s why the ocean was in Kansas and Illinois. And while the late Cretaceous transgressions and regressions are probably the best known, there were similar events during the early Cretaceous as well. Sea-level change graph by Robert Rhode and Global Warming Art, by way of Wikimedia. Used under Creative Commons license. So if, as I suggested the other day, we can’t call on glacial changes to account for these dramatic rises and falls of sea level, what is the cause? Tectonic activity, especially sea-floor spreading that produced mid-ocean ridges, was high during the Cretaceous, and is typically cited as a reason for the generally increasing sea level through the late Cretaceous, and reduced tectonic activity near the end of the Period might be a rationale for the dramatic regression, or drop in sea level, seen at and after the end of the Cretaceous. But those reasons are hard to use to explain the alternating rises and falls seen during the late Cretaceous. Maybe it was more specific tectonic events such as collisions and mountain building, which might take place over a few million years, and then they were done. Erosion then would start to actively send sediment into the oceans, perhaps in enough volume to displace enough water to increase sea level. Another tectonic way to increase relative sea level is to lower the land. Subsidence of the continents, induced by things like sediment loading and structural pushing of great slabs of rock on top of others, could have allowed the sea to transgress across the land. When we talk about the mountain building in western North America later this month, this idea will come into play I haven’t found any reports on a good correlation between specific tectonic events and the record of transgressions and regressions, but such work might be out there. If any listeners or readers know of such research, please let me know! Having an accurate record of sea-level changes is valuable in terms of our general understanding of earth history, of course, but it also has practical applications for oil exploration, as a predictive tool for knowing where in the sequence of rocks you might expect a beach sand, with good porosity as an oil reservoir, to be found. And there are also obviously implications for our understanding of modern climate change and sea levels. * * * Today’s geological birthday is Edward Salisbury Dana, born November 16, 1849, in New Haven, Connecticut. His father was the prominent geologist James Dwight Dana, but the son E.S. Dana made monumental contributions to the fields of mineralogy and crystallography. His books, the System of Mineralogy, Textbook of Mineralogy, and Manual of Mineralogy defined the way geoscientists look at minerals and crystals. Although revised, edited, and updated by others, Dana’s Manual of Mineralogy is still the basic text for mineralogy students today. —Richard I. Gibson Late Cretaceous transgressions Correlating transgressions and regressions Creta
You probably recall that sharks have been around since the Silurian, about 420 million years ago, or possibly even longer ago. They became abundant in the Devonian and expanded in diversity during the Carboniferous. It’s not true that sharks have remained unchanged in all that time, but the basic plan and structure of sharks has been generally about the same. The second major radiation of shark varieties, including stingrays and their kin, began during the Jurassic and continued in the Cretaceous, and most of the modern families of sharks date to the Cretaceous. Photo of Cretaceous shark’s tooth from Morocco by Parent Géry, used under Creative Commons license. Modern sharks and rays are in the group called neoselachians, which date to Permian time. They expanded in diversity during the late Mesozoic, possibly because of a contemporary expansion in small bony fishes which must have constituted much of the prey of Cretaceous sharks. Marine reptiles such as ichthyosaurs and plesiosaurs undoubtedly competed with sharks for the role of top predator in the Jurassic and Cretaceous oceans, but the marine reptiles went extinct at the end of the Cretaceous, while sharks survived. Because sharks have cartilaginous skeletons rather than the hard calcium phosphate of bones, body fossils are quite rare. Shark’s teeth are by far the most numerous fossils representing sharks, because they are harder and more resistant – the calcium phosphate mineral, apatite, like human bones and teeth. Some individual sharks grew hundreds or even thousands or tens of thousands of replaceable teeth over their lifetimes, so they are abundant in the fossil record in many places. In the United States, the deposits of the Cretaceous Interior Seaway in the west and Great Plains area, as well as the Cretaceous rocks of the Atlantic and Gulf Coastal Plains, contain many sharks’ teeth. A nearly complete, articulated specimen of a shark has been found in the Cretaceous of Kansas. —Richard I. Gibson Shark evolution Rise of modern sharks Hammerheads at the optometrist Photo of Cretaceous shark’s tooth from Morocco by Parent Géry, used under Creative Commons license.
Today, November 14, is the day the Precambrian would have ended if this calendar were at a proper time scale. And everything we have covered since February 1 would have to be squeezed into the next 6 weeks. That’s why I chose to organize the original book and these podcasts as I have, so I could give more time to each of the more recent periods. But geologic time, deep time, is really hard to grasp. I hope this helps a bit with that. * * * Geologic map of the US (from USGS) showing the Atlantic and Gulf Coastal Plains south and east of the heavy black line. The Cretaceous rocks that crop out in the most inland part of the plains (green colors) underlie the Cenozoic (Tertiary) rocks in orange and yellow, and the Cretaceous rocks continue in the subsurface far offshore as well. Our Cretaceous topic today is the Atlantic and Gulf Coastal Plans of the United States. These relatively flat lands are underlain at depth by all sorts of complicated geology, reflecting the various orogenies that were parts of the assembly of Pangaea, as well as the break-up to produce the present continents. But the rocks closer to the surface of these plains have been virtually undisturbed since they were laid down, beginning in the Cretaceous. The sedimentary rocks of the coastal plains are from times when sea level was higher. In Late Cretaceous time, the shoreline was located well inland from where it is today, crossing through east-central North Carolina, central South Carolina and Georgia, and central to northwestern Alabama. Almost all of what is now the state of Mississippi was under water, and there was a huge embayment that extended up what is now the Mississippi River valley all the way to southern Illinois. Southeast Arkansas, all of Louisiana, and a wide swath of eastern Texas were also under the Cretaceous sea. The positions of the shoreline in the Cretaceous, and later into the Cenozoic era, shifted many times. In our earlier discussions, that kind of transgression and regression of the sea was often attributed to glacial periods, alternately locking up water into ice sheets and melting. There’s no evidence for Cretaceous glaciation, so we have to call on some other rationale for the changing sea levels. Probably the most popular possible cause relates to changes in ocean water level because of increases and decreases in tectonic activity. More high-standing mid-ocean ridges would displace more water, raising sea level. But once established, the mid-ocean ridges were more or less still there over time, so that explanation doesn’t work too well. Sedimentation on the coastal plains and the adjacent continental shelves of eastern and southern United States has been more or less continuous for 100,000,000 years or more, making for a huge pile of sediment – as much as 30,000 feet in places. The sediments are mostly clastics, sand, silt, mud, and gravel, washed off the continent into the adjacent sea. This is continuing to this day, with the Mississippi River the leading source of sediment into the Gulf of Mexico. Throughout the US East Coast, a topographic break marks the boundary between the coastal plain sediments and the complex igneous and metamorphic rocks of the Appalachian Orogenic belt. It’s called the fall line, because the escarpment the boundary forms results in waterfalls in many places. The Great Falls of the Potomac River is one such waterfall. During colonial times, the fall line was typically the head of navigation for the coastal rivers, so cities like Richmond, Virginia, and Columbia, South Carolina, sprang up along it. All that material washing into the Gulf of Mexico, especially the porous sand, created a great many reservoirs for oil and natural gas. Both the Cretaceous and Tertiary sediments in the Gulf of Mexico hold many oil and gas fields. Although the general setting was similar on the east coast, there are no oil and gas fields there – probably because of differences in organic content of the source rocks, or in the
Base from NOAA (annotated by Gibson) It was the obvious good fit of the coastlines of Africa and South America that helped lead Alfred Wegener to his theory of continental drift back in 1915. The South Atlantic has a different history from the North Atlantic, not least in being rather younger than the northern portion. The geometry of the coasts reflects differences in the way parts of the South Atlantic opened. The near east-west margin of West Africa turns to north-south at the Gulf of Guinea, the corner where the northeastern tip of South America used to be attached. In fact, that corner is probably really a triple junction, a tectonic location where three relatively distinct rifts began, radiating away from the location of the Niger Delta today. The combined South America-Africa continent, which we’ve referred to previously as West Gondwana, began to separate in several places during the Jurassic. In the north, the irregular boundary between West Gondwana and North America left a piece of West Gondwana attached to North America – Florida. And the zone between West Africa and northern South America began to crack, too. In the south, also during the Jurassic, we talked last month about the separation of East Gondwana, and that also put the beginnings of a rift between southern Africa and southern South America. But the middles of what are now Africa and South America were still attached to each other until the Cretaceous. At the corner, today’s Gulf of Guinea, two pull-apart rifts started. One ultimately became the rift that makes up most of the South Atlantic, with the north-south margin of Africa from Gabon down to South Africa on one side and Brazil on the other. The other rift was within the African continent, extending northeast from today’s Niger Delta, practically all the way across Africa, through Chad and Sudan to the Red Sea. In places this was a pull-apart zone, especially in Nigeria, where it is called the Benue Rift, but further into Africa it is a tear or shear zone, with the northern and southern parts of Africa moving alongside each other. If this rift had not failed, we would have two continents today instead of one single African continent. Another shear zone developed in the third branch of the triple junction. This branch ran west from the Niger Delta today, along the east-west trending coast of West Africa, from Nigeria to Ghana to the Cote D’Ivoire to Liberia. On the southern side of this zone, the northern coast of Brazil, from the tip at Cabo San Roque up to the mouth of the Amazon, that section was sliding to the west. So the tectonic boundary between South America and Africa was mostly strike-slip faults, also called transform faults, where the two big blocks slid alongside each other and ultimately parted, leaving the Middle Atlantic Ocean in their wake. The southern Atlantic Ocean was formed in a more straightforward way, with the two sides pulling apart more or less perpendicularly from the spreading center at the Mid-Atlantic Ridge. But as usual, there was plenty of variety within those overall general parameters. In the southern, relatively simple rifted system, things were complicated by two hotspots that poured basalt and other volcanics into the widening ocean and on the adjacent continents as well. We talked about one of them, the Tristan Hotspot that produced the Parana Basalts and the Rio Grande-Walvis Seamount Chain, the other day. (November 11) The second one was further north, and today it is reflected in active volcanoes like Mt. Cameroon in Africa and St. Helena, the volcanic island near the Mid-Atlantic Ridge. Between them under the ocean, the St. Helena seamount chain represents the movement of the African Plate over the hotspot as the South Atlantic opened. And there is a conjugate submarine ridge on the South American side too. The big deal about these two volcanic centers, the Tristan and St. Helena hotspots, is that as the South Atlantic was opening, the volcanics erupted from
To start with today, I have a correction. In the episode for October 29, when I was talking about cycads, I misrepresented some research I cited. I said the study indicated that cycads had gone extinct and then re-emerged 10 million years ago, but the study was actually talking about a radiation, a rediversification, of cycads 10 million years ago, following a decline from the end of the Cretaceous. I’m sorry for this mistake, which has been corrected in the online blog post. Thanks to an anonymous commenter for pointing out my error. * * * Back to the Cretaceous. Drawing of Quetzalcoatlus feeding by Mark Witton and Darren Naish, used under Creative Commons license. We have to talk about pterosaurs again, because during the Cretaceous they became the largest flying animals in the history of the earth. Pterosaurs were reptiles, but not closely related to dinosaurs nor to the birds that were descended from dinosaurs. They were the first vertebrates to achieve true flight. They were really quite diverse, and not all of them were huge. The smallest known pterosaur is a specimen from the Jehol Biota of northeastern China, a not-quite-adult pterosaur with a wingspan of about 25 centimeters, or 10 inches. It lived in early Cretaceous time, about 120 million years ago. At the other end of the size spectrum, two specimens have been found that date to the last 4 million years or so of the Cretaceous Period, about 70 to 66 million years ago. Quetzalcoatlus was discovered in the Javelina Formation at Big Bend National Park, Texas in 1971. You’ll see estimates for its wingspan up to 16 meters or 52 feet, but the consensus today seems to be more like 11 meters or 36 feet – still huge, given that the largest wingspan of a modern bird is the albatross, at 3.5 meters, or 11 feet. Fossil remains of a late Cretaceous pterosaur found in Romania were described in 2002 with the genus name Hatzegopteryx, but there is a lot of opinion among researchers that it may be identical with Quetzalcoatlus. It had a similar wingspan. Because these fossils are quite fragmentary, it has been challenging to estimate the body weight of these huge pterosaurs. Some researchers speculate that they could not in fact fly, though I gather that that is a minority view. There’s even more debate about their mode of feeding. Some ideas suggest that they were skimmers, snagging fish out of the near surface of waterways and oceans, but others include the idea that they were actually quadrupedal on land. Most pterosaurs were. The bones of their wings, essentially their front legs, were strong enough for them to lumber around, and perhaps they did that as they scavenged land-based life. Arguments have been put forth against both the fish-eating and the scavenging modes of feeding, and a 2008 study suggests that they were terrestrial stalkers, preying on small land animals as modern storks do – bur remember that storks and other birds have no close affinities with pterosaurs – they just maybe occupy similar ecological niches. —Richard I. Gibson Drawing of Quetzalcoatlus feeding by Mark Witton and Darren Naish, used under Creative Commons license.
Much of the Mississippian limestone in southern Indiana is uniform in its color and texture, properties that make it an excellent building stone. It’s used in many monument facings too. The Empire State Building, National Cathedral, Chicago Public Library, Metropolitan Museum of Art, the new Yankee Stadium, and the roof of the immigration building at Ellis Island are all Indiana Limestone. 35 of the 50 state capitol buildings feature Indiana limestone. Sanders Quarry in Salem LimestoneThe rock is more technically known as the Salem Limestone, which formed in the warm, shallow Mississippian seas about 335 to 340 million years ago. The region was distant enough from land that very little detritus washed into the lime, so the resulting rock is more than 97% pure calcite, calcium carbonate. The rock is fossiliferous, but many of the fossils are of tiny, even microscopic animals called foraminifera. They are one-celled organisms that made calcareous shells. Most are no more than a millimeter across. From the building stone point of view, irregularities like fossils aren’t as important as the fact that the Salem Limestone has few bedding planes to disrupt the rock. It’s massive, so blocks of the limestone can be cut out for building stone and for decorative facings. Stone used for these purposes is called dimension stone in the industry, to differentiate it from crushed stone used for things like aggregate in concrete. The rock has been quarried since 1827, and by the 1920s, something like 80% of the limestone quarried in the United States for dimension stone came from southern Indiana, mostly around the towns of Bloomington and Bedford. There are still 9 active quarries in the area. * * * Today’s birthday is George Gaylord Simpson, born June 16, 1902, in Chicago. He was a prominent and influential paleontologist who contributed greatly to evolutionary theory regarding the details of how evolution takes place. He spent most of his career at Columbia and Harvard and the University of Arizona. —Richard I. Gibson Reference: Indiana limestone Sanders Quarry photo by Sphinxcat via Wikipedia (public domain).
I’ve said for months that Gondwana is coming toward southeastern North America. It’s still coming, but the remaining ocean between the two continents was really pretty narrow by middle Mississippian time. The Supercontinent of Gondwana included South America, Africa, Arabia, India, Antarctica, and Australia, plus a few additional bits. One of the most interesting bits, from North America’s point of view, was a triangular zone between what is now west Africa and northern South America, along the Venezuelan coast. That triangular zone included what is now Florida and southern Georgia and parts of Alabama and the Bahamas. It was absolutely part of Gondwana at this time, but fear not, we’ll get it fairly soon. North America was tilted relative to its present geography, so that the Mississippian equator ran more or less through the middle of the continent, with what is now the east coast forming a southern coastal edge to the continent. The northeastern part of North America – the Maritime Provinces of Canada, Newfoundland, Labrador, and Greenland, were still pretty firmly attached to Europe by the remnants of the Caledonian Mountains, even though they were fairly old by Mississippian time. The central part of Europe, France, central Germany, and points east, were assembling as a result of small continental blocks that had been shed off the northern flank of Gondwana colliding with the margins of Baltica. What’s now the Iberian Peninsula, Spain and Portugal, was involved in a complex mountain belt called the Variscan Orogeny, which won’t really culminate until the late Carboniferous and Permian Periods, 20 to 50 million years after the end of the Mississippian or early Carboniferous. * * * On June 15, 1991, Mt. Pinatubo on Luzon Island in the Philippines reached the climactic phase of a multi-day eruption. Pinatubo had been inactive in recent memory until the 1991 events, and the mountain was deeply eroded and supported extensive forests and human populations. The eruption ejected huge quantities of ash, and the reported death toll of about 900 was largely the result of buildings collapsing under the weight of the ash. Evacuations saved thousands, but the lives of pretty much everyone in the region of the eruption were severely disrupted. The volume of ejected material was the second greatest in the 20th century, estimated to be a bit less than the eruption at Katmai, Alaska, in 1912, which we discussed a week ago. The ash and especially the aerosols Pinatubo put into the atmosphere did have global climatic impacts, reducing the average global temperature by almost a degree Fahrenheit or a half degree Centigrade. —Richard I. Gibson Early Mississippian map Reference: AAPG Memoir 43, Evolution of the Arctic-North Atlantic and the Western Tethys, by Peter A. Ziegler (1988). U.S. Geological Survey Photograph of Pinatubo eruption in 1991 taken by Richard P. Hoblitt.
First, my disclaimer about the time scale of this calendar of earth history. It’s not at a proper scale. If it were, we’d be in the Precambrian until mid-November. So I’ve arbitrarily assigned the Precambrian to January, the Cenozoic Era to December, and the months between are the periods of the Paleozoic and Mesozoic Eras. It’s June, and that means we’re in the Mississippian Period. We’ve talked a bit about chert before, but today’s short episode is to say a little more about it. Chert, fined-grained impure silica, SiO2, appears in sedimentary rocks in two ways. It can form when silica-rich waters percolate through the rocks after they have solidified, with the chert deposited in openings within the rock. And it can be an original part of the rock, deposited at the same time as the rest of the sediment. RadiolariaChert does appear in sedimentary rocks earlier in the Paleozoic era, but it’s really quite rare until the Mississippian Period. Tiny animals called radiolarians secrete siliceous shells. Radiolarians span the entire time from Cambrian to present, but they may have increased in abundance during the Mississippian so that their skeletal remains could have contributed to the increased abundance of chert in the Mississippian rock record, but I’m not sure if that is the explanation for Mississippian chert. Radiolarian remains today on the deep ocean floor cover large areas with siliceous ooze, soft sediment made mostly of radiolarians and diatoms, algae that also make siliceous shells. Siliceous ooze on the ocean floor is probably chert in the making. It will take many tens of thousands of years, maybe even millions of years, for the ooze to be buried by additional sediment, for the water to be driven off, and for it to lithify into chert. Chert comes in a wide variety of colors. It’s often black, but it can be brown, yellow, reddish, and even white. The colors reflect impurities incorporated into the silica, including organic matter and iron. —Richard I. Gibson Image from Kunstformen der Natur (1904), by Ernst Haekel, via Wikipedia
In the Grand Canyon area, the rocks equivalent to the Madison Limestone that we talked about yesterday are called the Redwall Limestone. It forms one of the highest near-vertical cliffs in the canyon, as much as 500 to 800 feet high. The Redwall is a typical gray to tan limestone – so why is it called Redwall? It is stained red by erosion of the overlying rocks, especially the Supai group and Hermit Shale, which is red because of oxidized iron in the shale. The shale was deposited in a low mud flat that was periodically exposed to air and at other times underwater, which allowed the iron in the rock to oxidize. Shale erodes quite easily so it washed down the cliff faces and stained the underlying Redwall. Redwall Cavern, Grand Canyon Fairly soon after the deposition of the Redwall – and this applies to the Madison limestone in many places, too – gentle uplift brought the limestones above sea level. Then the action of moving water, percolating through the rock, did what it does to limestone – it dissolved out caves. In a good number of places, caves collapsed, and sinkholes and other features typical of karst topography developed. This was a pretty widespread development, because we can see the evidence of the collapses in the Black Hills, in Montana, and in the top of the Redwall limestone too. This was not remotely related to the cave formation that made the modern Lewis and Clark Caverns we talked about yesterday, which are at most a couple million years old, but was something that happened probably quite soon after these Mississippian limestones were lithified. How do we know that? Because the collapse structures don’t include much of the overlying rock layers. The collapsing had to take place before those layers were laid down. I can assure you that the Redwall does make really steep cliffs. Probably the most difficult hike of my life was on a perfectly smooth, level trail about three or four feet wide along the Redwall’s face. The problem was there was a drop-off on the left that went straight down about 400 feet, and the wall on my right went straight up another 400 feet. If you’re interested in the travelogue of that 1987 backpack trip, here’s a link to my report on it. —Richard I. Gibson Photo in Redwall Cavern, Grand Canyon, by Richard I. Gibson
The Madison Limestone of the Rocky Mountains and Great Plains is formally a geologic group that includes several formations, including the Lodgepole Formation and Mission Canyon Formation. It was formed in those warm, shallow Mississippian seas that covered a lot of the interior of North America about 330 to 340 million years ago. The calcium carbonate deposited in those seas accumulated to as much as 2,000 feet thick in parts of Montana, where the Madison forms prominent gray cliffs. The Gates of the Mountains, named by Lewis and Clark for dramatic cliffs along the Missouri River east of Helena, Montana, are made of these Mississippian limestones. Madison Limestone at Gates of the MountainsThe Madison was named back in 1893 for outcrops along the Madison River near Three Forks, Montana, or perhaps for the nearby Madison Mountain Range, but it has equivalents with different names as far away as the Black Hills of South Dakota, central Colorado, and Arizona. It was an extensive shallow sea. You probably won’t be surprised to hear that the warm shallow water supported lots of life. The Lodgepole Formation especially has loads of fossils, ranging from crinoid stem columnals to brachiopods and small horn corals and much more. The Lodgepole Formation has nice bedding sometimes distinguished by a little silt or mud interbedded or mixed with the limestone. The beds are often two to four inches thick and sometimes show color differences that give the rock a distinct striped appearance – but don’t visualize bright colors, we’re talking about shades of tan and gray. The Mission Canyon formation, younger than and on top of the Lodgepole Formation, is massive. That’s a technical term that means we seldom see the discrete beds like the ones in the Lodgepole, or for that matter in most layered sedimentary rocks. The Mission Canyon is just limestone. Lots of it. Microscopically, the Mission Canyon is called bioclastic – that means “life, broken” – and the rock is often made up mostly of tiny broken pieces of shells and crinoid stems and such, all cemented together by more calcite. It’s usually gray, and because it’s thick and doesn’t have planes of weakness, bedding planes, the Mission Canyon sometimes makes really prominent cliffs like those at the Gates of the Mountains. Limestone is easily soluble in rainwater, which is normally slightly acidic because of the reaction between water and carbon dioxide in the atmosphere, which makes carbonic acid. Even in arid country like Montana, caves can develop. Lewis and Clark Caverns, a state park along the Jefferson River east of Whitehall, Montana, probably formed when Montana was a lot rainier than it is today, probably during the glacial periods of the past couple million years. Lewis and Clark were on the river just below the caverns, but they never saw them. The caves were discovered by non-Native Americans in 1882, and they were designated our second National Monument in 1908, but because the park service couldn’t manage it, the cave was transferred to the State of Montana in 1938 and became Montana’s first state park. The porous, easily dissolved limestones of the Madison Group serve as important oil reservoirs in places like the Williston Basin of eastern Montana and western North Dakota, and elsewhere they are valuable groundwater aquifers. —Richard I. Gibson Photo of Madison Limestone at Gates of the Mountains by Richard I. Gibson.
During the Mississippian, a special kind of carbonate build up evolved, called Waulsortian Mounds. They were not reefs – they did not contain the kinds of organisms like corals that could build large complex structures. You may recall that the end-Devonian extinction decimated the reef-building organisms, and we don’t find true reefs in the geologic record for a hundred million years after the Devonian extinction. These mounds are the only constructions known during the interval until reef builders recovered. They are not simple piles of sediment – they can reach 200 meters in height with very steep flanks. They seem to really be constructions of some kind. And they are found in a restricted geographic setting – the southern margin of the Laurussian Continent, formed by the collision of North America and Baltica, the core of Europe. Today, this zone is in present-day Belgium – the community of Waulsort, Belgium, gives its name to these mounds – southern England, Wales, and Ireland, Illinois, Missouri, and Kansas, and north up to North Dakota and Alberta. During the Mississippian, that margin was very near the paleo-equator, so the mounds probably relate to processes and life in warm, tropical seas. The entire southern margin of Laurussia was probably a relatively narrow but continuous ocean, in part the Tethys Ocean between Gondwana and Eurasia and in part the ocean that remained between Gondwana and southeastern North America. Waulsortian mounds are mostly lime mud, including some broken skeletal fragments of things like crinoids and bryozoans. It is not clear exactly why the mounds formed, but there may be a connection to the likely storm tracks of hurricanes at the time they formed in the Mississippian Period. Or more specifically, the lack of storms. The mounds seem to be in regions that would likely have been protected from the most common tracks of hurricanes, so that they would have had time to grow by whatever mechanism. David King, at Auburn University, shows a connection between the mounds and low wave energy, another way of suggesting that major storms were rare or absent. I have a link on the blog to King’s interesting report on Waulsortian mounds. Were these mounds constructed by life processes such as sediment binding by bacteria, or by some inorganic process of selective cementation? As near as I can tell, the answer is not certain. It may have been a combination of processes, made possibly by the fortuitous absence of large storms. —Richard I. Gibson Link: David T. King, Jr.’s report
The Tri-State lead-zinc district lies around the common corner of Missouri, Kansas, and Oklahoma. Lead and zinc were produced from Mississippian rocks that historically were called the Boone Formation, but have been subdivided and given other names including Reeds Spring, Keokuk, and Warsaw formations. The rocks that host the lead-zinc deposits are mostly limestones that were deposited in warm, shallow seas, the more or less standard for middle America during the Mississippian Period. It’s not 100% limestone – there’s a lot of chert, fine-grained silica, interbedded with it and as nodules in the limestone, as well as some shale in places. The mineralization appears to be related to faults and fractures in the limestone, complicated by dissolution and collapse of the limestone in some places. The fractures provided abundant pores and passages for mineral-rich waters to flow through, but the ultimate origin of those fluids is debated. The most common view is probably that the mineral-rich fluids rose from some deep, magmatic source until they were stopped by an impermeable layer and found the open fractures in which to crystallize the lead and zinc minerals. Sphalerite photo by Rob Lavinsky, iRocks.com CC-BY-SA-3.0The deep magma from which the hot mineral-rich water rose might have been related somehow to the flank of the Ozark Uplift, which got its start as long ago as the Ordovician, but there was some ongoing, relatively gentle tectonic activity over many millions of years, including during the Mississippian. As is often the case, it’s difficult to pin down the time the minerals came in with accuracy. It has to be younger than the rocks that contain the minerals, of course – they and their fractures had to be there first. And there’s good evidence that the deposition of minerals must have taken a long time, probably in many episodes, possibly spanning as much as many tens of millions of years. The most common minerals are galena, lead sulfide, and sphalerite, zinc sulfide. The minerals were so important to the early history of this region there’s even a town in Kansas named Galena. Mining began about 1848 and continued until the Eagle-Pitcher Mine in Oklahoma shut down in 1967. Over the century from 1850 to 1950, the district produced about half the Zinc mined in the United States and 10% of the lead. One mine complex, the Pitcher Field in Oklahoma, was the most prolific producer, yielding about 60% of the total which amounts to more than 15 million tons of lead and zinc over the history of the mining district. —Richard I. Gibson Resources: Report from Oklahoma Historical Society Report from Kansas Geological Survey part 1; part 2 The Geology and Ore Deposits of the Tri-State District: D.C. Brockie et al., in Ore Deposits in the United States, John D. Ridge, ed., American Inst. Of Mining, Metallurgical and Petroleum Engineers, 1968, p. 400. Sphalerite photo by Rob Lavinsky, iRocks.com – CC-BY-SA-3.0
With all the talk about limestone, you might get the impression that all of America was a big beautiful tropical seaway with nice white carbonate sand everywhere. While the shallow seas were extensive, there were other kinds of sediments deposited as well. In what is now the eastern Great Lakes, Ohio, Kentucky and West Virginia, during early Mississippian time some huge river deltas formed, as large as the Mississippi or Nile Delta today. from USGS Prof. Paper 259Remember that the Great lakes weren’t there – it would be hundreds of millions of years before they would form. The complex upland in eastern North America, the result of collisions with Baltica and other pieces of Europe, led to a huge river system in what is now southern Ontario. The Ontario River flowed south, into the eastern edge of the sea that covered most of the United States west of the Appalachians. The river carried a lot of sand, silt, and mud into the branch of the sea called the Ohio Bay, and built a long linear delta complex, a peninsula that extended all the way from present-day Lake Erie to the border between Kentucky and West Virginai – almost three hundred miles long and something like 75 miles wide. The sediments in the delta are known today as the Bedford Shale and Berea Sandstone. There was another peninsula to the west, in west-central and southwestern Ohio, but this one wasn’t a delta. It was all that was left of the Cincinnati Arch – the broad upland that got its start 130 million years earlier, during the Ordovician. As the Mississippian Period progressed and sea levels became higher and higher, the peninsula sometimes called Cincinnatia was reduced to a string of islands and shoals and eventually was pretty much completely submerged. West of Cincinnatia, in present-day Indiana and Illinois and points west, most of the Mississippian rocks are the shallow-water limestones with abundant fossils that are so common from this time across much of North America. The Berea Sandstone and Bedford Shale represent oscillations in sea level that produced variations in the sedimentation pattern over time at various places. Modern deltas do this too, changing the position of the main channel and smaller distributary streams sometimes on an annual basis. So you can get coarse river channel sands at one time, but muds from floods over the banks of the channels at other times in the same place, and many variations in between. Deltaic systems can make pretty good reservoirs for oil and natural gas when coarse, porous sandstones are encased within impermeable fine-grained shales. And in fact the Berea Sandstone was historically an important oil and gas producer in Ohio. —Richard I. Gibson Reference: Geology of the Bedford shale and Berea sandstone in the Appalachian basin, USGS Prof. Paper 259 (1954) Also source of map above.
Just a short one today, to mention the brachiopods again. Two of the major groups of brachiopods, the productids or spiny brachiopods, and the sprifers, with elongate, wing-like shells, continued to thrive during the Mississippian. Productus longispinus (left); Spirifer glaber (right) In 2008 a report by Chinese and Polish paleontologists described an unusual assemblage of silicified brachiopods, including 4 new species, from the Muhua area of southern China. LINK * * * Laki Eruption Beginning on June 8, 1783, and continuing for eight months, a fissure – a crack in the earth – and 130 eruptive vents began to pour basaltic lava over southern Iceland at Lakagigar, often called Laki. By most estimates the volume of erupted material, more than 14 cubic kilometers, is the greatest on record during historic times. The lava was accompanied by poisonous fumes composed of hydrofluoric acid and sulfur dioxide which killed half of all the livestock in Iceland and devastated the countryside well beyond the lava flows themselves. The resulting famine killed an estimated 25% of Iceland’s human population. Worldwide, the ash and acid aerosols in the atmosphere caused dramatic climatic effects – crop failures in Europe, droughts in India, extremely low flows in the river Nile, and a North American winter cold enough for the Mississippi River to freeze over at New Orleans. The sulfurous fumes were strong enough in France to kill a few dozen people, and the U.S. ambassador to France, Benjamin Franklin, speculated on the connections between the poisonous fogs in Europe and the Icelandic eruption – correctly, as it turned out. The total death toll worldwide from this eruption may have been as high as 6 million when all the impacts of the famines are included. There’s a new book out on the Laki eruption, just published in March 2014. Island on Fire is by Alexandra Witze, an award-winning science journalist. I haven’t finished it yet, what I have read is an excellent report on the eruption and its worldwide consequences. —Richard I. Gibson Brachiopod drawing from an old textbook (public domain)
The Mississippian Period was much like the earlier part of the Paleozoic Era in North America – much of the continent was covered by warm shallow seas in which abundant life contributed to the thick sheets of limestone that were laid down. Eastern North America was still a highland, the result of the ongoing collisions with Europe and the microcontinents that were rifting away from Gondwana, and the ocean between North America and Gondwana was getting narrower and narrower. In western North America, the Antler uplift that we discussed at the end of May was still there, and it was shedding sediment into the western seaway. The combined weight of those sediments and the piles of thrusted rocks pushed over the western edge of North America by the Antler Orogeny pushed the earth’s crust downward, creating what’s called a foreland basin. The Antler foreland basin extended through what is now eastern Nevada, western Utah, and into parts of Idaho and California. It was a relatively deep trough, and the mud that found its way into it contained a lot of organic material, washing in from both the west and the east. The resulting rock is called the Chainman Shale – a rock we mentioned in the Devonian, in May, as the source rock for the oil fields of Nevada. The Chainman is an excellent source rock, as much as 8% total organic carbon in some places. There was some tectonic activity in what is now Alaska’s North Slope and across the Arctic islands of northern Canada – it’s called the Innuitan or Ellsmerian Orogeny and it was mostly taking place toward the end of the Devonian and into the Mississippian Period. In Europe, Gondwana was pretty much encroaching on the southern margin of Baltica, but it was a complex interaction with lots of smaller blocks colliding. The seaway between Gondwana and Europe, called the Tethys Sea, is actually still there. We call it the Mediterranean Sea today. —Richard I. Gibson Links to Paleogeographic maps: North America Western North America Europe Article: Innuitan Orogeny