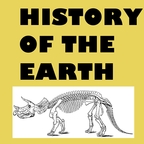
History of the Earth
Summary: 366 snapshots of earth history in the form of a perpetual calendar, with daily episodes for 2014 and weekly thereafter. Find all the posts at http://historyoftheearthcalendar.blogspot.com
- Visit Website
- RSS
- Artist: Richard I. Gibson
- Copyright: Copyright Richard I. Gibson
Podcasts:
What is dynamic topography? Well, it depends on who you ask. Dynamic topography is similar to other terms, like uplift, that have been used in so many different ways that you really have to look at the document you’re reading to understand what the author is talking about. This term has been applied to places around the world, like the Colorado Plateau in the United States, South Africa, the Aegean, and East Asia, which makes it even more complicated to tease out its meaning. Most broadly, dynamic topography refers to a change in the elevation of the surface of the earth in response to something going on in the mantle. This “something” can include both the flow of the mantle, as well as differences in mantle temperature or density. For the purposes of this podcast, I will use a more strict definition: Dynamic topography is the change in the elevation of the surface of the earth in response to the upward or downward flow of the mantle. How much higher or lower can dynamic topography make the earth’s surface? Well, that’s a matter of debate. Earlier studies have suggested that several kilometers, or over 6000 feet of modern elevations can be explained by things going on in the mantle. More recent work instead suggests that dynamic topography creates changes of at most a three hundred meters, or a thousand feet. A good example of a place where this process is thought to be active is Yellowstone. As Dick Gibson discussed in the December 19th, 2014 episode, Yellowstone is thought to be a hot spot. That is, an area of the earth where hot material moves from deep within the mantle to the base of the crust, causing significant volcanism at the surface of the earth. Other well-known hot spots are located in Hawaii, and Iceland. So how can a hot spot like Yellowstone cause dynamic topography? Well, you’ve probably seen a similar process at play the last time you played in a pool or a lake. Think of the surface of the pool like the surface of the earth. If you start moving your hands up and down under water, the surface of the pool starts to move up and down. If you ever tried to shoot a water gun upwards underwater when you were a kid, you probably remember it pushing up the surface of the water, and being disappointed that it didn’t shoot out at your friend or sibling. As an adult, you could try holding a hose upwards in a pool. Again, it probably won’t shoot out, but will gently push upwards on the surface of the pool. Dynamic topography concept. © Commonwealth of Australia (Geoscience Australia) 2017, used under Creative Commons Attribution 4.0 International Licence The principle for a hot spot creating dynamic topography is the same. The flow of the mantle pushes upwards, warping the crust and increasing the elevation of the earth’s surface above the hot spot. Near Yellowstone, this results in an area of high elevation which lies next to the Snake River plain. But Dynamic Topography doesn’t just cause increases in elevation, it can also pull the earth’s surface downward. In North America, dynamic topography is thought to have been in part responsible for the creation of the Cretaceous interior seaway. As a reminder, the Cretaceous interior seaway was a shallow sea that covered parts of western North America, in middle to late Cretaceous time, about 100 to 79 million years ago. Its size varied, but at its greatest extent the seaway stretched through Texas and Wyoming in the US, and Alberta and to the Northwest Territories in Canada. It was widest near the US-Canadian border, where it stretched from Montana to western Minnesota. Low elevations in western North America that allowed the ocean to flood in and form this shallow sea may have been caused by downwards flow in the mantle. This downwards flow was likely caused by oceanic crust that was subducted at the western margin of North America. That is, oceanic crust that went underneath the North American plate and into the mantle. Because this crust was par
Today’s topic is three minerals with the same chemical formula: Kyanite, Andalusite, and Sillimanite. How can three things with the exact same chemical formula, Al2SiO5, be different minerals? Many of you probably recall that besides a distinct chemical composition, a mineral has a definite crystalline structure. And these three minerals each have completely different crystallography. The basic reason for the different crystal structure is that the chemicals aluminum and silicon, arrange themselves differently depending on conditions of pressure and temperature. Kyanite forms at relatively low temperatures over a wide range of pressures while sillimanite crystallizes at relatively high temperatures, generally above 700º C over a similar range of pressures to kyanite. Andalusite develops in a more limited temperature-pressure field, call it medium temperatures but always relatively low pressures. All that variety happens under metamorphic conditions, when rocks are undergoing lots of changes such as those that happen when continents collide, or when subduction scrunches some parts of the crust against others. So that means these minerals are usually found in metamorphic rocks, and in fact they are called index minerals for the particular conditions that they represent. Kyanite is probably the most familiar of the three. It’s often a beautiful blue color, making long, lath-like crystals, so it’s popular with collectors. Kyanite also has a nearly unique, and diagnostic property. Whereas most minerals have a particular hardness, kyanite has two. On the Mohs hardness scale, kyanite is 5 in the direction along the length of the crystals, but 7 across them. Together with the color and crystal habit, this makes kyanite pretty easy to identify. Andalusite and sillimanite are less common. But andalusite also makes interesting crystals, especially when carbon gets included in the growing crystals. That can produce a distinctive elongate four-armed cross, a variety called chiastolite that is sometimes polished to make jewelry. Sillimanite certainly can also make nice crystals, but I guess I’ve led a sheltered life, or maybe I just haven’t mapped enough metamorphic rocks. I’ve never seen a large sillimanite crystal in the wild, just fibrous, wispy, almost feathery coatings in metamorphic rocks like schist and gneiss. So these things are cool collectible minerals and they help geologists figure out the pressures and temperatures that formed rocks, helping unravel the geologic history of the places where they are found. But they also have economic value. Kyanite and andalusite especially are mined to make mullite, another aluminum silicate that’s pretty rare in the natural world but pretty common as a synthetic material made from kyanite. Toilet bowls, which you might call porcelain, are more or less mullite. Most of it is made from a clay mineral, but kyanite can be added to improve its toughness and stability. And small amounts of kyanite go to making abrasives in things like automobile brake shoes. But by far the greatest use of kyanite and andalusite is in making mullite for refractories – ceramics that retain their strength and remain chemically inert at very high temperatures. Furnaces, kilns, and crucibles in the iron and steel industries are often constructed with mullite bricks, and steel making consumes something like 70% of all the aluminum silicates produced worldwide. The United States is the world leader in producing kyanite. It’s mined at four places in Alabama and Georgia, where the metamorphic rocks of the Appalachian Mountains contain abundant reserves. US mine production of kyanite, at about 100,000 metric tons a year, is more than we need, so we export about a third of what we produce – one of only a handful of mineral commodities that the US is self-sufficient in. The total value is around $30 million a year. South Africa produces more andalusite than the US produces kyanite, so it’s the world leader in producing
For today’s episode of the podcast I’m introducing you to Dr. Petr Yakovlev, a friend and geologist here in Butte at the Montana Bureau of Mines and Geology. Petr will be doing occasional guest episodes to give you all a break from my voice, as well as information about some of the diverse things he's worked on. Petr Yakovlev with a Cenozoic conglomerate near Cardwell, Montana. Photo by Dick GibsonPetr got his undergrad geologic education at Boston College and his PhD at the University of Michigan. In this episode Petr and I talk about his work in Tibet, which has implications for the fundamental nature of the India-Eurasia collision; another structural geology project he worked on in the Catskills of New York; and the projects he’s working on here in Montana. And he gives us some teasers about the kinds of topics he plans to cover for History of the Earth. Running time 13 minutes. .
Today’s Episode takes us to Australia, the home of the Riversleigh Lagerstätte. Lagerstätten, you may recall, are fossil assemblages that typically have extraordinary diversity as well as extraordinary preservation. The Riversleigh Lagerstätte fits that definition well, containing fossils of 15- to 25-million-year-old mammals, including an extinct giant platypus, as well as birds, reptiles, frogs, and lungfish. But the story begins about 500 million years ago, not 25 million years ago. In Early to Middle Cambrian time, more than half a billion years ago, the supercontinent of Gondwana was pretty much assembled, with South America, Africa, India, Antarctica, and Australia comprising a wide, long continent that stretched from the South Pole to north of the Equator. The prong of the continent including Australia was the part that was in the tropics, and much of what is now northern Australia was covered by a warm, shallow sea. In what is now northwestern Queensland, where the Riversleigh Lagerstätte is found, the shallow sea transgressed and regressed – meaning it came and went – and among other things, sediments included grainy shallow-water limestones. The resulting Thorntonia Limestone contains fossils that I’m sure are interesting and informative, including trilobites, brachiopods, and stromatolites, pretty typical of Cambrian rocks. But that’s not the lagerstätte. Nimbadon, a koala-like tree-dweller. From Wikipedia.Fast forward about 475 million years, to the Oligocene epoch of the Paleogene Period of the Cenozoic Era. The rocks of northern Queensland were dissolving in a warm, wet, tropical environment, and the limestones of the Thorntonia formation were developing karst topography – caves and surficial pools rich in dissolved calcium carbonate. Starting about 25 million years ago, near the end of the Oligocene, animals began to die in those pools and become trapped in the caves, where ongoing calcium carbonate deposition preserved them remarkably well. So even though the origin of the calcium carbonate was a really ancient rock, the Cambrian Thorntonia formation, it was the much more recent dissolution of those rocks that provided the material that preserved the fossils at Riversleigh. Ages of the fossils extend from late Oligocene time into Miocene, around 15 million years ago, but there are younger fossils as well since the caves are still there and deposition is continuing. The Riversleigh site is not just one location, but many, spread out over a hundred-square-kilometer area that was named a World Heritage Site in 1994. Riversleigh contains the richest assemblage of bat fossils in the world – at least 35 different species. Besides bats, mammal fossils include extinct koalas, marsupial lions, wombats, herbivores the size of sheep, at least 14 species of opossum, and 15 different kangaroo species. While many fossils are remarkably well preserved, some, such as Yalkaparidon, are only known from a few teeth and scattered bones, making their relationships to other families uncertain. In fact, some are grouped colloquially as Thingodonta, meaning “toothed thing”. Some researchers have suggested Yalkaparidon was a mammalian woodpecker. Bird fossils at Riversleigh range from extinct flightless rails to storks and lyrebirds. Reptiles are represented by tree-dwelling crocodiles, horned turtles, and dragon lizards, which were probably related to iguanas. There are even snakes and frogs and two species of lungfish, all of which are really rare in the fossil record, especially as long ago as the Riversleigh assemblage, 15 to 25 million years. Some lagerstätten record essentially an instant in geologic time, or a relatively short period. An example of such an assemblage is the fish of the Green River Formation in Wyoming, many of which may have been killed in a single event – a heavy fall of ash into the lake where they lived, from an erupting volcano. So Riversleigh, in addition to its diversity and excellent preservation
Today for episode 381, we’re going to Zealandia. No, it’s not a quirky TV show modeled after Portlandia. It’s the 7th largest continent on earth. We’re not talking continents in the geographic sense, really large land masses like Africa and South America, but we are talking about continental material in the geologic sense, even though most of it is submerged beneath the ocean. Public Domain via WikipediaZealandia is centered on New Zealand, and in some ways, it’s been known for decades, ever since good charts of subsurface bathymetry were created. Those data show a complicated mix of rises and troughs on the sea floor around New Zealand, including the Lord Howe Rise in the Tasman Sea between New Zealand and Australia, the Campbell Plateau east of New Zealand, and other highs and lows. Although the ocean floor around the globe is diverse, with seamounts and trenches, fault zones and piles of volcanic rocks here and there, on the whole oceanic crust is deep and uniform, or at least varying broadly and predictably. These relatively small-scale high plateaus on the ocean floor around New Zealand are unusual. Let’s define a continent. Geographically, it usually means a large land mass. “Large” is subjective, but Australia, at about 9 million square kilometers, is considered to be the smallest continent. And the count depends on whether you separate Europe from Asia, or just say Eurasia as one, and other conventions. Many would say that a degree of isolation and separation also defines a continent, so Eurasia should be one, together with Africa, North America, South America, Antarctica, and Australia, for a total of six. Forget all that. We’re looking at places made of continental crust, the generally lighter, silica-rich material in contrast to oceanic crust, denser and more iron-rich. By that geologic definition, all the conventional continents really are mostly continental crust too. All well and good. There are numerous smaller, separated areas of continental crust that are usually called microcontinents. Madagascar is probably the best example of a microcontinent, but there are lots of them, and you can get into plenty of arguments about whether a piece like, say, Greenland, is or is not fully a part of the North American continent. So, let’s go to Zealandia. In 2017, Nick Mortimer and colleagues, writing in GSA Today, pretty much laid any argument to rest. There’s abundant evidence to say that there is a large, diverse terrain centered on New Zealand but 94% submerged, that is mostly continental crust. It adds up to about 5 million square kilometers, comparable in size to Arabia and India, sub-continents that are connected in one way or another with full-fledged continents. Zealandia is close to, but clearly separated from Australia, and Mortimer and others make a convincing case that it should be considered the seventh continent, six times larger than Madagascar, the biggest microcontinent. So if continental rocks are lighter, which accounts for them being for the most part above sea level, why is most of Zealandia under water? The crust there is thinner, so it doesn’t rise as high as that on most continents, and it has been stretched and broken, partly rifted apart during a long complex history. Zealandia was probably originally part of the supercontinent of Gondwana, attached to eastern Australia and West Antarctica, when those two continents were fully attached to each other about 105 million years ago, in Early Cretaceous time. That margin of Gondwana, thousands of kilometers long, was a subduction zone somewhat like today’s Andes, with an old Pacific oceanic plate diving beneath the Gondwana continental crust. There would have been a volcanic mountain range there, recorded in Zealandia as a surviving string of granitic rocks, batholiths similar to those that developed in western North and South America during Mesozoic and Cenozoic time and continuing to this day. Gondwana began to be rifted apart by about 85 million yea
The badlands at Makoshika State Park are in Montana, and so am I, but Montana’s big. The park is just outside of Glendive, almost at the eastern border of the state. It took me and my friend about seven hours to get there from Butte, but it was worth it. Eastern Montana is quite different from western Montana, geologically. The west is broken, thrusted, pulled apart, and intruded, while the east is – with exceptions – largely more or less flat-lying, relatively undisturbed sedimentary rocks piled upon each other, with the oldest at considerable depth and the younger rocks at or near the surface. At Makoshika, the rocks are from the last part of the Cretaceous Period and the early part of the Cenozoic Era, what we used to call the Tertiary. So the famous K-T boundary, the extinction point for the dinosaurs and a lot more, is in these rocks. Nowadays we call the earliest period of the Cenozoic the Paleogene, and if you’re really interested in the story of the names of the Cenozoic periods, check the podcast episodes from early December 2014. Erosional features in the Fort Union Formation at Makoshika. Photo by Dick Gibson.There’s actually a coal bed near the K-T boundary at Makoshika, but the coolest thing about the rocks in my opinion is their weird and fanciful erosional shapes. The younger Tertiary rocks, the Fort Union Formation, are alternating sandstones and siltstones and shales, with a wide range of cementation, so the rocks respond to erosion very differently. Some erode easily while others stand out in rugged relief. Erosion creates pedestals and balanced rocks, natural bridges and sharp ridges, as well as softer slopes incised by rivulets large and small when it rains. The sediments that came to make up the Fort Union Formation were deposited in rivers, lakes, and swamps around 65 to 60 million years ago, Paleocene time. There’s a bit more about the Fort Union Formation in the episode for December 4, 2014, which you can find on the blog, history of the earth calendar.blogspot.com, together with transcripts for this and older episodes. The Fort Union Formation’s rocks included many highly vegetated swamps which over time dehydrated and were compressed into thick coal beds, among the most important coal producers in the United States today. U.S. demand for coal is decreasing, as cheaper, more abundant fuels for generating electricity, most notably natural gas, are used more, but in 2017 burning coal was still the source for about 30% of US electricity. Just ten years ago, that proportion was close to 50%. Coal mines in the Fort Union Formation in Wyoming, south of Makoshika, produce about 40% of all US coal. The Cretaceous rocks underlying the Fort Union Formation are similar kinds of rocks, but they contain dinosaur bones, especially further west, and they’re called the Hell Creek Formation. Triceratops and Tyrannosaur fossils have been found in the Cretaceous rocks at Makoshika, along with a nearly complete Thescelosaurus, a small, 10-foot-long probable herbivore discovered in 1997. The name Makoshika is from the Lakota words maco sica, meaning 'bad land' or 'land of bad spirits,' but despite that I find the land remarkably beautiful. One of the reasons the landscape displays such spectacular erosional features is the fact that this area is gently uplifted. The Cedar Creek Anticline, a long, linear fold above a deep-seated fault trending northwest through this part of southeastern Montana, reaches its northern end near Makoshika. You’d never think of this as a mountain uplift, but older, more erodible rocks of the Hell Creek Formation have been warped to the surface here, where wind and water have sculpted them for many thousands of years. Features like natural bridges and balanced rocks and delicately carved monuments are ephemeral in geologic terms, usually surviving for hundreds or a few thousand years at most. But they are continually replaced by other features, until the rocks with their variable resistance are
Today’s episode, number 379, is about glaciers in what is now the Sahara Desert, and we’re going back 340 million years, to the Mississippian or Early Carboniferous Period of the Paleozoic Era. In the original daily episodes of this podcast back in 2014, when we got to the Mississippian in June, I had a very brief episode about glaciers in Australia and South America. They probably represented an early pulse of the well-known later glacial period during the Permian, and the glaciation provided evidence for the existence of the supercontinent of Gondwana, which was situated more or less over the south pole at that time, about 340 million years ago. Gondwana consisted of the continents and smaller blocks we know today as South America, Africa, Arabia, Antarctica, India, and Australia, and the pole was located somewhere in south-central Africa, so it should come as no surprise that some recent work by Daniel Le Heron at the University of London, published in the journal Geology in November 2017, reports evidence for an extensive ice sheet in what is now northern Chad, in the modern Sahara Desert. Permian Gondwana reconstruction and inferred ice cap (blue outline) from A. du Toit, South African geologist, 1937 (Our Wandering Continents). Du Toit's work was remarkably prescient. The map above differs only slightly from modern reconstructions of Gondwana. The red oval shows the general area where Le Heron (2017) infers a small ice sheet in Carboniferous time. Le Heron described belts of sinuous lineaments carved by ice in belts five to 12 kilometers wide and spread over an area of more than 6,000 square kilometers. The features cut into an ancient surface that is interpreted to represent the landscape over which the glaciers flowed during that Early Carboniferous time. He suggests that the ice sheet extended to the west to cover much of what is now Niger, and that the glaciers flowed northward into the sea. The coastline then was also in far northern Chad, so the glaciers probably reached the ocean. The present-day surface of the earth in northern Chad is actually an exhumed, an uncovered, ancient landscape that formed about 340 million years ago when those glaciers scoured the land. The surface was buried by later sediments which have since been eroded away. I don’t think the causes for this glacial episode are well understood, although there’s been a lot of work done on it. One of the most recent reports, by Yves Goddéris & Yannick Donnadieu and their colleagues writing in Nature Geoscience in April 2017, suggests that the onset of glaciation was the result of tectonic activity. Just before the ice age got going, the Hercynian Mountains had been uplifted because of continental collisions in many parts of the world. As soon as mountains are uplifted, they begin to erode, and a lot of erosion tends to reduce atmospheric carbon dioxide because there’s a lot more material, the sediment, to react with the CO2. Goddéris and his co-workers think CO2 levels fell enough to trigger the formation of glaciers in the mountains, and ultimately by Permian time, to form extensive ice sheets that covered a huge area of Gondwana. According to their model, the glacial period ended when the mountains had been eroded enough to affect the carbon cycle and allow CO2 levels (and overall temperatures) to rise again. The end of glaciation was also likely affected by the final amalgamation of the supercontinent of Pangaea, which changed climates to more extensive arid conditions. If you are interested in more about the Mississippian or Early Carboniferous Period, search the archives for June 2014. All 30 episodes that month were about the Mississippian.—Richard I. Gibson Le Heron paper Goddéris paper
If you’ve listened to this series for any length of time, you know that geologists, like most scientists, are fond of jargon. They use specialized words as shortcuts for particular meanings, like ‘gabbro,’ which is easier to say than “a coarse-grained igneous rock with considerable iron and magnesium, often dominated by the minerals pyroxene and plagioclase.” I try to explain jargon terms in this podcast when I use them, since my goal really is to help understanding. Today’s jargon word is pegmatite. It’s from a Greek word meaning “to stitch together,” and the crystals in pegmatites often do show a complex interlocking texture. But the key thing about pegmatites is that the crystals in them are big. Sometimes really big. There isn’t really a legal definition in geology for “big,” but pegmatites usually have crystals bigger than one or two centimeters, and some would use 2.5 centimeters, one inch, as an arbitrary cut-off. But it isn’t just size that matters. Pegmatites often, but not always, have a more or less granitic composition, so they often contain large crystals of quartz and especially feldspar. But they often form late in the history of an igneous granite body, and because those late-stage magmas contain superheated water, they can mobilize and concentrate elements that solidify into minerals at relatively low temperatures as the molten granite cools. Minerals containing lithium, boron, tantalum, and rare earth elements are pretty common in pegmatites. The crystals grow to large sizes because they have longer times to grow – that’s the basic difference between rocks like granite and rhyolite, which have essentially the same composition, but granite cools slowly and has large crystals while rhyolite cools more quickly and has a very fine-grained texture. Think of pegmatites as the slowest of all, slow enough that in some cases crystals tens of feet long can form. At the Etta Pegmatite in the Black Hills of South Dakota, spodumene, a lithium silicate, grew into huge, log-like crystals up to 42 feet long. The mine there was an important source of lithium for years. Tourmaline is another fairly common mineral, actually a group of minerals, found in pegmatites. It’s a complex boron silicate that often makes long, black rod-like crystals, but it’s sometimes beautiful green, pink, and other colors, even sometimes zoned from inside to outside like a watermelon. Tourmaline group minerals are hard, around seven on the Mohs harness scale, so the gemmy colored varieties are sometimes made into jewelry. Besides their value as sources for large, collectible mineral specimens, pegmatites in some places are valuable economic resources, like the spodumene containing lithium I mentioned a minute ago. Rare earths, beryllium, and tantalum are often found in pegmatites, ultimately finding their way into things like cell phones, automobile brake shoes, and capacitors in computers. In addition to containing large crystals, pegmatites can be big themselves. Some of the pegmatites in South Dakota are more than a mile long, but just last fall I visited a little one in the hills east of Butte, Montana, where I live. That one was no more than a meter, three feet, across, but it did have pretty cool feldspar crystals in it more than six inches long. —Richard I. Gibson Pegmatites of the Black Hills
Today’s topic for Episode 377, the Tepuis of South America, was suggested long ago by a listener. Photo of Mt. Roraima by Jeff Johnson, used under Creative Commons Attribution-Share Alike 3.0 Unported license.Transcript: The Tepuis are huge, high-standing plateaus isolated from their surroundings by near-vertical cliffs. The name means "house of the gods" in the language of the Pemon, the indigenous people who live in the region of northeastern South America where the tepuis are found. They’re especially numerous around the common borders of Venezuela, Brazil, and Guyana, and they include Mount Roraima, the setting for Arthur Conan Doyle’s 1912 novel “The Lost World”—a nearly inaccessible, remote, high, jungle-covered terrain. Doyle imagined the isolated preservation of dinosaurs and other extinct critters in his novel. You commonly see the tepuis called the oldest plateaus on Earth, with suggestions that they are two billion years old. This is absolutely untrue: the rocks are indeed ancient, but the plateaus themselves as landforms are vastly younger, and there aren’t any dinosaurs – sorry. We’ll talk about both geological aspects of this unique ecosystem, the rocks and the landforms they make. The area is part of the Guyana Shield, one of the ancient cores of the South American continent called cratons, from a Greek word for strength. Cratons make up the hearts of all the continents. In North America, multiple pieces of somewhat different age underpin most of Canada, with the Superior Craton extending into Minnesota, Wisconsin, and Upper Michigan. South America is made up of two large ancient cratons, the larger one in central and coastal Brazil, and the other, the Guyana Shield, in Venezuela and the Guianas and adjacent parts of northern Brazil. The rocks that form the near-vertical escarpments of the tepuis were laid down as diverse sandy sediments probably about 1900 to 1500 million years ago, early to middle Proterozoic time. Some of the rocks that make up the Guyana shield are even older, back into Archaean time, more than two and a half billion years old, but they generally underlie the tepuis rather than form them. All these ages are similar to the cratons of the other continents. Ancient Precambrian rocks have usually undergone multiple episodes of tectonic activity, burial, and heating, so they are mostly metamorphic rocks, which means changed form from their original sedimentary nature. The high cliffs that form the walls of the tepuis are mostly quartzite, not much different from the sand they originally were, and they are among the youngest of the Precambrian rocks in the Guyana Shield. They’re still relatively flat lying, not highly contorted like many ancient rocks, and because they are resistant, that helps them stand high and uneroded. But not completely unerodable. These quartzites even hold caves whose origin is not well understood. That brings us to the formation of the plateaus themselves. That probably happened really very recently geologically speaking, as the result of erosion. The 6,000 feet of erosion that formed the Grand Canyon happened in just the past 5 or 10 million years or so, just yesterday, geologically, even though the rocks there are hundreds of millions to more than a billion years old. The cliffs of the tepuis are high, but not as high as the Grand Canyon is deep. Angel Falls, the highest in the world, drops off Auyan Tepui almost a thousand meters, 3200 feet. The entire tepui is somewhat higher, about 1,600 meters, almost at one mile or 5,200 feet. So the erosion that carved these cliffs, spectacular though they are, could be quite recent, like the Grand Canyon. There is some evidence that the plateaus might be older than that, but still much, much younger than the rocks that form them. I’ve seen some suggestions that the tepuis formed as erosional plateaus as long ago as 70 million years. It’s almost impossible for me to believe that, because in what has been an a
Welcome to the History of the Earth podcast where we discuss all things geological. I’m your host, geologist Dick Gibson. There’s been a long hiatus in my production of this podcast, and I hope to rectify that with some new episodes. Today’s topic for Episode 376 is U.S. mineral dependency. It’s a common misconception, perhaps an expression of “American exceptionalism,” that the United States is self-sufficient in most or all of the mineral commodities we use in our stuff every day. Nothing could be further from the truth. In my book, What Things Are Made Of, published in 2011, I documented the uses for everything from arsenic to uranium, and where the U.S. gets its supply of each. At that time the U.S. was self-sufficient, a net exporter, of only 19 of the eighty-plus commodities the U.S. Geological Survey tracks. Today, in 2017 the situation is no better – the count is down to 15 items for which the U.S. is a net exporter. Some of those items are low-value but interesting things like boron, kyanite, and diatomite. Only three, gold, iron ore, and molybdenum, are high-value metals. A lot of the mineral commodities we import are obscure, but the vast majority of technologically active Americans use them every day. For example, indium, critical to making flat-panel displays for televisions and computers, is all imported, mostly from Canada and China. Zinc is a well-known metal used mostly in galvanizing iron to prevent rust and in brass and bronze for everything from door knobs to saxophones. In the U.S., 82% of it is imported, mostly from Canada and Mexico. What about that vital bomb-making element, uranium? Hardly any is used to make bombs in the United States any more, but it helps generate electricity in 61 commercial nuclear power plants across the nation, and almost 20% of our electricity comes from nuclear plants. Where does that uranium come from? Only about 4% of it is mined in the United States. The rest is imported, with Canada and Kazakhstan providing close to half, and Russia, Australia, and Namibia supplying most of the rest. How about something as common as a flashlight battery? Dry-cell batteries are made with zinc, carbon, and a pasty electrolyte of ammonium chloride and manganese dioxide. All the manganese used in the U.S. is imported, with more than two-thirds of the manganese ore we use coming from Gabon in Central Africa. By far most of the nearly 700,000 tons of manganese the U.S. consumes goes to steel alloys, where it helps make the steel resistant to abrasion and stronger in impacts. That makes it a common alloy in bicycle frames and mining tools. Or consider common salt. Even though there are 64 plants in 16 states, with Kansas leading the way in production, the United States still imports about a quarter of all the salt we use. About half the salt consumed goes to highway deicing, but a third or so is used to make a wide variety of chemicals, including plastics like polyvinyl chloride or PVC. Food processing and common table salt amount to just 3% of the salt used in the U.S. Chile is the largest source for salt imports, with Canada and Mexico second and third. The point of my book What Things Are Made Of and this brief set of examples is simply to help you recognize the profound level of globalization that exists in everyday products Americans use. Thanks for listening, and I hope you’ll join us next time for another episode of the History of the Earth. —Richard I. Gibson
Garnet is the birthstone for January, but there really isn’t a single mineral named ‘garnet.’ It’s a group of at least 15 minerals of differing chemistry, but only six are common. They are three aluminosilicates combined with iron, magnesium, or manganese, and three calcium silicates in combination with either iron, aluminum, or chromium. The definition of a mineral is something with a specific chemistry and a definite crystalline structure. All six garnet minerals have essentially identical crystal structure. They are in the isometric or cubic crystal system, meaning their three crystal axes are perpendicular and the same length – that’s what isometric means, same length. But garnets seldom form cubes. The molecules are of sizes that result in many-sided forms, including dodecahedrons, with 12 faces, and trapezohedrons with 24 faces, and more complicated shapes, all of which tend to make garnets almost spherical. Often, they look like little soccer balls in the rock. The word garnet is from an early English word that meant dark red, as many garnets are, but it may ultimately be derived from the words pomum granatum, Latin for pomegranate – because pomegranates have bright red seed covers similar in appearance to common garnets. And they’re pretty. Red, purple, yellow, brown, orange, bright green for uvarovite, the chromium garnet – and they’re hard, usually around 7 or higher on the Mohs hardness scale. This makes them great for two things: gemstones and abrasives, and they’ve been used for both since the Bronze age. By far the greatest use of garnets, by volume, is as abrasives. The US uses about 190,000 tons of garnets every year, with about 17% of that mined in New York’s Adirondack Mountains and in Idaho. The rest, 83% in 2014, was imported mostly from Australia and India and a bit from China. India produces about half the world’s industrial garnet, with China second with a third and Australia 15%. The US is a distant fourth with only 2% of world production. You benefit from garnets every day, even if not directly in the rough surface of an emery board or sandpaper. Waterjet cutting is used to cut and shape metals from steel to aluminum as well as plastics and glass. Besides their use as abrasives, about a fifth of garnet consumption in the United States goes to filtration in water wells. The value of industrial garnet in the US is about $9 million. Garnets are used for gemstones, but because they are so common, their value is nothing like that of diamonds, sapphires, rubies, and others, most of which are also harder than garnet and much rarer. But garnets can still make beautiful jewelry. Do I have any garnets in my collection? Yup. Hundreds of thousands of them, but most of them are microscopic, in a rock with a blue mineral called glaucophane from the California Coast Ranges. The biggest one I have is maybe an inch or so across, from Ontario, but at Gore Mountain, New York, there are garnets as much as two feet across in what is possibly the largest garnet deposit in the world. They are in metamorphic rocks related to a continental collision about a billion years ago, called the Grenville Orogeny. So happy birthday to everyone with a January birthday. Have a garnetiferous day! —Richard I. Gibson
Running time 3 hours We are up to the Cenozoic Era in the monthly episodes. This one combines the 31 episodes from December 2014, covering the Cenozoic, into one episode, and it completes the packaging of each month of episodes from the original series. As usual for this monthly compilation, I’ve left the references to specific dates in the podcast so that you can, if you want, go to the specific blog post that has links and illustrations for that episode. They are all indexed on the right-hand side of the blog. Thanks for your interest in this project. If you have questions or comments, please let me know, either here on the blog – there’s a page for Questions– or contact me by email at rigibson at earthlink.net. I’ll try to respond. You can of course also leave a review on iTunes. I really do appreciate your feedback. For 2016, I’m going to TRY to have more frequent new programs than I did in 2015. Some upcoming topics, many suggested by you listeners, include fluorescence in minerals, questions about the oxygen crisis back in the Precambrian, the Tepuis in South America, and more. Thank you! —Richard I. Gibson
Running time 2 hours 30 minutes We are up to the Cretaceous Period of the Mesozoic Era in the monthly episodes. This one combines the 30 episodes from November 2014, covering the Cretaceous, into one episode. As usual for this monthly compilation, I’ve left the references to specific dates in the podcast so that you can, if you want, go to the specific blog post that has links and illustrations for that episode. They are all indexed on the right-hand side of the blog. If you have questions or comments, please let me know, either here on the blog – there’s a page for Questions – or contact me by email at rigibson@earthlink.net. I’ll try to respond. You can of course also leave a review on iTunes. I really do appreciate your feedback. —Richard I. Gibson
This week, our topic is twins. Twinned crystals. When two, or sometimes more, crystals of the same mineral share volumes or surfaces within the crystal lattice, they grow together in interesting ways. One common kind of twin is essentially a reflection across a plane, a plane that is shared by both crystals. They can end up growing perpendicular to each other, or at a specific angle in what are called contact twins. One common type of contact twin is in the common mineral quartz, silicon dioxide, when two crystals grow at almost right angles to each other, sharing a common plane. This kind of twin is called a Japan Law twin because they are found frequently in quartz crystals from Japan, although they were first described from localities in the French Alps. Sometimes multiple crystals grow together. Aragonite, calcium carbonate, crystallizes in the orthorhombic crystal system, which means it has three unequal but perpendicular crystal axes that form the basis of the molecular crystal lattice. Think of a shoe box, with three different edge lengths all at right angles to each other, that’s an orthorhombic crystal lattice. But in aragonite, sometimes particular planes within the crystals serve as common surfaces for crystallographically distinct crystals, and they grow together to form near-perfect hexagons. This can be confusing when you’re trying to identify a mineral, like aragonite, which you know is not hexagonal but there you have these nice hexagons. They’re multiple twins, actually composed of three separate crystals of aragonite grown together in a pseudohexagonal form. The other primary type of twin is called a penetration twin, where two distinct crystals share not just a plane, but an entire volume within the combined twin crystal. They end up looking like the two crystals penetrate each other. Probably the most famous example of a penetration twin is the mineral staurolite, an iron-aluminum silicate. It’s also orthorhombic and usually forms little box-like crystals, typically like the shoe box but with one dimension often a lot thinner than the thinnest dimension of a shoe box. If two crystals share the center of the box, you end up with twins that look for all the world as if one crystal of staurolite has penetrated through the other. You can get two different angles in this sharing that make forms that look like crosses. The two crystals can cross at a 60-degree angle or at 90 degrees, making what are called cruciform – cross-shaped – twins. Such twins in staurolite are relatively common. Fluorite, calcium fluoride, is another mineral that often forms beautiful penetration twins. Fluorite is isometric, meaning it has three perpendicular but equal crystal axes. In terms of fluorite crystals, this means that instead of that shoe-box shape, you often get perfect cubes where all the edges have the same length. If two cubes grow together and share a volume of molecules, you get twins that can be pretty cool. There are quite a few different twin laws, and they really are “laws” to the extent that these kinds of intergrowth can only follow specific patterns, all controlled by the geometry of the crystal and the size and arrangement of the molecules in it. Thanks for your interest! —Richard I. Gibson
Running time 2 hours 5 minutes We are up to the Jurassic Period of the Mesozoic Era in the monthly episodes. This one combines the 31 episodes from October 2014, covering the Jurassic, into one episode. As usual, I’ve left the references to specific dates in the podcast so that you can, if you want, go to the specific blog post that has links and illustrations for that episode. They are all indexed on the right-hand side of the blog. If you have questions or comments, please let me know, either here on the blog – there’s a page for Questions– or contact me by email at rigibson at earthlink.net. I’ll try to respond. You can of course also leave a review on iTunes. I really do appreciate your feedback. —Richard I. Gibson